LFI design, qualification, and performance
Contents
Overview[edit]
The Planck-LFI instrument is an array of 11 radiometric receivers in the Ka, Q and V bands, with centre frequencies close to 30, 44 and 70 GHz. The exact centre frequencies for each receiver are reported in [A. Mennella, M. Bersanelli, R. C. Butler et al. Planck early results. III. First assessment of the Low Frequency Instrument in-flight performance. A&A, 536:A3, 2011]; for simplicity, here we will refer to the three channels using their nominal centre frequency. A detailed description of the LFI instrument is given in [M. Bersanelli et al. Planck pre-launch status: Design and description of the Low Frequency Instrument. A&A, 520:A4, 2010], and references therein.
The LFI description[edit]
The LFI instrument (see Figure 1 below) consists of a 20 K focal plane unit hosting the corrugated feed horns, the orthomode transducers (OMTs) and the receiver front-end modules (FEMs). Fourty four composite waveguides [O. D’Arcangelo, L. Figini, A. Simonetto et al. The Planck-LFI flight model composite waveguides. Journal of Instrumentation, 4:2007, 2009] are interfaced with three conical thermal shields and connect the front-end modules to the warm (~300 K) back-end unit (BEU) containing a further radio frequency amplification stage, detector diodes and all the electronics for data acquisition and bias supply.
Best LFI noise performance is obtained with receivers based on InP High Electron Mobility Transistor (HEMT) low noise amplifiers (LNAs) for minimal power dissipation and best performance. To further minimise power consumption in the focal plane, the radiometers are split into two sub-assemblies connected by waveguides, one located at the telescope focal area, the other on the 300 K portion of the Planck satellite. These design features allow the entire front-end LNAs dissipation to be <0.55 W, which enables the active cooling of the focal assembly. This is achieved with a vibration-less hydrogen sorption cooler, which also provides 18 K pre-cooling to the HFI helium J-T cooler. Two sorption cooler units are included in the flight hardware.
As shown schematically in Figure 2 below, the LFI consists of the following subsystems:
- Radiometer Array Assembly (RAA)
- Sorption Cooler Subsystem (SCS)
- Radiometer Electronics Box Assembly (REBA)
The RAA includes the Front End Unit (FEU) and the Back End Unit (BEU), connected via waveguides. The FEU is located at the focus of the telescope, as one component of the joint LFI/HFI focal assembly (see sections below). The BEU is mounted on the top of the Planck SVM. The Radiometer Electronics Box Assembly (REBA) and the warm parts of the Sorption Cooler System (SCS) is located on one of the lateral panels of the service module (SVM). The FEU and the Sorption Cooler Compressor (SCC) are connected by concentric stainless steel tubes. The smaller tube carries hydrogen at ∼60 atmospheres from the cooler compressors to the FEU, while the larger tube returns the hydrogen at ∼0.3 atmospheres. These units are described in detail in following sections, the SCS is described in details in the satellite section dedicated to Cryogenics. All LFI units are linked together by the LFI harness, which also connects to the spacecraft interface.
Radiometer Array Assembly (RAA)[edit]
The Radiometer Array Assembly (RAA) consists of two main units (the front end unit, FPU and the Back End Unit, BEU), connected by a set of waveguides. The Focal Plane Unit (FPU) is the heart of the LFI instrument and it contains the feed array and associated orthomode transducers (OMTs) and FEMs, all cooled to 20 K by the sorption cooler. The FPU comprises a set of 11 modules, which are mounted on a mechanical support which meets the thermo-mechanical requirements of the instrument and adds thermal inertia. The BEU comprises the radiometer Back End Modules (BEM) and the Data Acquisition Electronics (DAE), which are connected by an internal harness. The HFI Unit is located inside the LFI FPU and supported by the LFI structure. The LFI structure gives the mechanical and thermal interface to the HFI unit with the proper stiffness and thermal de-coupling. The LFI structure also guarantees the proper alignment of the HFI detector with the telescope focal plane.
The time scale of the stability of the receiver is driven by the 1 rpm rotation speed of the spacecraft, which requires a very low 1/f-noise or gain variation of the low noise amplifiers and other components. The LFI uses a pseudo-correlation receiver concept (Figure 3 below). This radiometer concept is chosen to maximise the stability of the instrument by reducing the effect of non-white noise generated in the radiometer itself. In this scheme, the difference between the inputs to each of the chains (the signal from the telescope and that from a reference black body respectively) is continuously being observed. To remove the effect of instability in the back-end amplifiers and detector diodes, it is necessary to switch the signal detected at the diodes at high rate. The signals from the sky and from a reference load are combined by a hybrid coupler, amplified in two independent amplifier chains, and separated out by another hybrid. The sky and the reference load power can then be measured and differenced. Since the reference signal has been subject to the same gain variations in the two amplifier chains as the sky signal, the true sky power can be recovered. The differencing receiver greatly improves the stability if the two input signals are almost equal, at a cost of a factor of in sensitivity compared to a perfectly stable total-power radiometer with the same noise temperature and bandwidth. This radiometer concept is capable of greatly reducing the knee frequency. We define as Radiometer Chain Assembly (RCA, see Figure 3) each functional unit from the feed horn to the BEM. The RAA therefore includes a set of 11 RCAs and the Data Acquisition Electronics, all mounted on a suitable mechanical structure. Although there are differences in the details of the radiometer chains at different frequencies their overall configuration is similar, and a general description of its design is provided in this section. Planck LFI has 11 Radiometer Chain Assembly (RCA). Each RCA is constituted by feed horn and FEM in the FEU (at 20 K), BEM (at 300 K) in the BEU and four waveguides that connect each FEM-BEM couple. The frequency distribution of the RCA is the following:
- 2 RCAs at 30GHz
- 3 RCAs at 44GHz
- 6 RCAs at 70GHz
- Radiometer Chain Assembly (RCA);
Every radiometer chain assembly (RCA) consists of two radiometers, each feeding two diode detectors (see Figure 3 below), for a total of 44 detectors. The 11 RCAs are labelled by a numbers from 18 to 28 as outlined in Figure 1, right panel.
Figure 3 provides a more detailed description of each radiometric receiver. In each RCA, the two perpendicular linear polarisation components split by the OMT propagate through two independent pseudo-correlation differential radiometers, labelled as M or S depending on the arm of the OMT they are connected to (Main or Side, see lower-left inset of Figure 3).
In each radiometer the sky signal coming from the OMT output is continuously compared with a stable 4 K blackbody reference load mounted on the external shield of the HFI 4 K box [L. Valenziano, F. Cuttaia, A. De Rosa et al. Planck-LFI: design and performance of the 4 Kelvin Reference Load Unit. Journal of Instrumentation, 4:2006, 2009.]. After being summed by a first hybrid coupler, the two signals are amplified by ~30 dB, see upper-left inset of Figure 3. The amplifiers were selected for best operation at low drain voltages and for gain and phase match between paired radiometer legs, which is crucial for good balance. Each amplifier is labelled with codes 1, 2 so that the four outputs of the LNAs can be named with the sequence: M1, M2 (radiometer M) and S1, S2 (radiometer S). Tight mass and power constraints called for a simple design of the Data Acquisition Electronics (DAE) box so that power bias lines were divided into five common-grounded power groups with no bias voltage readouts; only the total drain current flowing through the front-end amplifiers is measured and is available to the house-keeping telemetry (this design has important implications for front-end bias tuning, which depends critically on the satellite electrical and thermal configuration and was repeated at all integration stages, during on-ground and in-flight satellite tests). A phase shift alternating between and at the frequency of 4096 Hz is applied in one of the two amplification chains and then a second hybrid coupler separates back the sky and reference load components that are further amplified and detected in the warm BEU, with a voltage output ranging from -2.5 V to +2.5 V.
Each radiometer has two output diodes which are labelled with binary codes 00, 01 (radiometer M) and 10, 11 (radiometer S), so that the four outputs of each radiometric chain can be named with the sequence: M-00, M-01, S-10, S-11.
After detection, an analog circuit in the DAE box removes a programmable offset in order to obtain a nearly null DC output voltage and a programmable gain is applied to increase the signal dynamics and optimally exploit the ADC input range. After the ADC, data are digitally downsampled, requantised and compressed in the radiometer electronics box assembly (REBA) according to a scheme described in [J. M. Herreros, M. F. Gómez, R. Rebolo et al. The Planck-LFI Radiometer Electronics Box Assembly. Journal of Instrumentation, 4:2008, 2009; M. Maris, M. Tomasi, S. Galeotta et al. Optimization of Planck-LFI on-board data handling. Journal of Instrumentation, 4:2018, 2009.] before preparing telemetry packets. On ground, telemetry packets are converted to sky and reference load time ordered data after calibrating the ADU samples into volt considering the applied offset and gain factors.
To first order, the mean differential power output for each of the four receiver diodes can be written as follows [M. Seiffert, A. Mennella, C. Burigana et al. 1/f noise and other systematic effects in the Planck-LFI radiometers. A&A, 391:1185–1197, 2002; A. Mennella, M. Bersanelli, M. Seiffert et al. Offset balancing in pseudo-correlation radiometers for CMB measurements. A&A, 410:1089–1100, 2003; M. Bersanelli, N. Mandolesi, R. C. Butler et al. Planck pre-launch status: Design and description of the Low Frequency Instrument. A&A, 520:A4, 2010]:
where
is the total gain, is the Boltzmann constant, the receiver bandwidth and is the diode constant. and are the average sky and reference load antenna temperatures at the inputs of the first hybrid and is the receiver noise temperature.The gain modulation factor [A. Mennella, M. Bersanelli, M. Seiffert et al. Offset balancing in pseudo-correlation radiometers for CMB measurements. A&A, 410:1089–1100, 2003; A. Zacchei, D. Maino, C. Baccigalupi et al. Planck early results. V. The Low Frequency Instrument data processing. A&A, 536:A5, 2011], , is defined by:
and is used to balance (in software) the temperature offset between the sky and reference load signals and minimise the residual 1/
noise in the differential datastream. This parameter is calculated from the average uncalibrated total power data using the relationship:where
and are the average sky and reference voltages calculated in a defined time range. The white noise spectral density at the output of each diode is essentially independent from the reference-load absolute temperature and is given by:
In Figure 4 below we show a close-up of the two front end modules of an RCA with the four phase switches which are labelled with the four letters A and B (main arm), C and D (side arm). Each phase switch is characterised by two states: state 0 (no phase shift applied to the incoming wave) and state 1 ( phase shift applied) and can either stay fixed in a state or switch at 4 kHz between the two states.
Phase switches are clocked and biased by the DAE and their configuration can be programmed via telecommand. In order to simplify the instrument electronics, phase switches are configured and operated in pairs: A/C and B/D. This means that if phase switches A and C are switching at 4 kHz then B and D are fixed both in the same state (either 0 or 1). This simplification, required during the design phase to comply with mass and power budgets, comes at the price of loosing some setup redundancy.
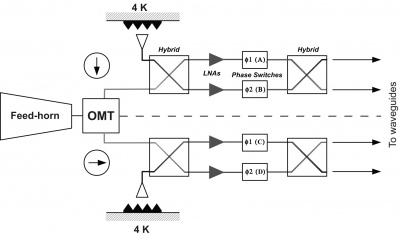
- Feed Horns (FH)
- OrthoMode Transducers (OMT)
- Front End Modules (FEM)
- Waveguides
- Back End Modules (BEM)
- 4K Load
REBA[edit]
Instrument On-board Software[edit]
Instrument Operations[edit]
LFI Ground Tests[edit]
During its development, the LFI flight model was calibrated and tested at various integration levels from sub-systems [R. J. Davis, A. Wilkinson, R. D. Davies et al. Design, development and verification of the 30 and 44 GHz front-end modules for the Planck Low Frequency Instrument. Journal of Instrumentation, 4:2002, 2009] to individual integrated receivers [F. Villa, L. Terenzi, M. Sandri et al. Planck pre-launch status: Calibration of the Low Frequency Instrument flight model radiometers. A&A, 520:A6, 2010] and the whole receiver array [A. Mennella, M. Bersanelli, R. C. Butler et al. Planck pre-launch status: Low Frequency Instrument calibration and expected scientific performance. A&A, 520:A5, 2010]. In every campaign we performed tests according to the following classification:
- Functionality tests, performed to verify the instrument functionality.
- Tuning tests, to tune radiometer parameters (biases, DC electronics gain and offset, digital quantisation and compression) for optimal performance in flight-like thermal conditions.
- Basic calibration and noise performance tests, to characterise instrument performance (photometric calibration, isolation, linearity, noise and stability) in tuned conditions.
- Susceptibility tests, to characterise instrument susceptibility to thermal and electrical variations.
Where possible, the same tests were repeated in several test campaigns, in order to ensure enough redundancy and confidence in the instrument behaviour repeatability. A critical comparison, that is central to the subject of this work, is the one between the results of on-ground and in-flight test campaigns. A matrix showing the instrument parameters measured in the various test campaigns is provided in Table 1 of [A. Mennella, M. Bersanelli, R. C. Butler et al. Planck pre-launch status: Low Frequency Instrument calibration and expected scientific performance. A&A, 520:A5, 2010].
The ground test campaign was developed in three main phases: cryogenic tests on the individual RCAs, cryogenic tests on the integrated receiver array (the so-called radiometer array assembly, RAA) and system-level tests after the integration of the LFI and HFI instruments onto the satellite. The first two phases were carried out at the Thales Alenia Space - Italia laboratories located in Vimodrone (Milano, Italy) (note that receiver tests on 70 GHz RCAs were carried out in Finlad, at Yilinen laboratories), system level tests (SLT) were conducted in a dedicated cryofacility at the Centre Spatiale de Liege (CSL) located in Liege (Belgium).
In Table 1 below we list the temperature of the main cold thermal stages during ground tests compared to in-flight nominal values. These values show that system-level tests were conducted in conditions that were as much as possible flight-representative, while results obtained during RCA and RAA tests needed to be extrapolated to flight conditions to allow comparison. Details about the RCA test campaign are discussed in [F. Villa, L. Terenzi, M. Sandri et al. Planck pre-launch status: Calibration of the Low Frequency Instrument flight model radiometers. A&A, 520:A6, 2010] while the RAA tests and the extrapolation methods are presented in [A. Mennella, M. Bersanelli, R. C. Butler et al. Planck pre-launch status: Low Frequency Instrument calibration and expected scientific performance. A&A, 520:A5, 2010].
Cryogenic system-level tests were split into three parts:
- Thermal balance, to validate the overall thermal mathematical model. The ground vacuum test equipment simulated the space environment.
- System cryogenic test, to check and optimise the satellite and instrument performance working at nominal temperatures.
- Thermal cycling test, to check the reliability of all electronics equipment and instruments to temperature variations (note that to allow the thermal control of the Service Vehicle Module (SVM), the warm part of the spacecraft was surrounded by a cryogenic shroud at a temperature lower than 100 K. The temperature of the shrouds was adjustable in order to allow the thermal cycling of the SVM during the tests. The temperature ranged between the two extreme values of C to C).
During the various test campaigns the instrument was switched off and moved several times in a time period of about three years. A series of functional tests were always repeated at each location and also in flight, in order to verify the instrument functionality and the response repeatability. No failures or major problems have been identified due to transport and integration procedures.
LFI In-flight Calibration[edit]
The Planck cryo-chain [Planck Collaboration. Planck early results. II. The thermal performance of Planck. A&A, 536:A2, 2011] is composed by three coolers: a 20 K Hydrogen sorption cooler, which is passively pre-cooled by the LFI focal plane and pre-cools the HFI 4K cooler; a Stirling 4K cooler that cools the HFI box and feed-horns and provides a 4K blackbody reference signal to the LFI receivers; a 0.1 K dilution cooler, which is pre-cooled by the 4K cooler and cools the HFI bolometer filters to K and the HFI bolometer detectors to ~0.1 K. The cooldown of the HFI 4K stage, in particular, was key during CPV for the LFI as it provided a variable input signal that was exploited during bias tuning.
V-groove radiator, cools theThe LFI CPV started on June, 2009 and lasted until August, when Planck started scanning the sky in nominal mode. At the onset of CPV, the active cooling started when the radiating surfaces on the payload module reached their working temperatures (~50 K on the V-groove, and ~40 K on the reflectors) by passive cooling. This was achieved during the transfer phase. Nominal temperatures were achieved on July, 2009, when the dilution cooler temperature reached 0.1 K [Planck Collaboration. Planck early results. II. The thermal performance of Planck. A&A, 536:A2, 2011; P. Meinhold, R. Leonardi, B. Aja et al. Noise properties of the Planck-LFI receivers. Journal of Instrumentation, 4:2009, 2009] (see Figure 5 below).
The CPV was carried out in four phases (see Table 2 below for a summary of the overall CPV test campaign):
- LFI switch on and basic functionality verification,
- Tuning of front-end biases and back-end electronics,
- Preliminary calibration tests,
- Thermal tests.
LFI Performance[edit]
Instrument Scientific Performance[edit]
Instrument Technical Performance and Budgets[edit]
(Planck) Low Frequency Instrument
LFI warm electronics Back End Unit
High Electron Mobility Transistor
(Planck) High Frequency Instrument
LFI Radiometer Array Assembly
Sorption Cooler Subsystem (Planck)
LFI Radiometer Electronics Box Assembly
LFI cryogenic amplifying stage Front End Unit
Service Module
Sorption Cooler Compressor assembly
Focal Plane Unit
LFI warm electronics Back End Module
LFI Data Acquisition Electronics
revolutions per minute
LFI Radiometer Chain Assembly
LFI cryogenic amplifying stage Front End Module
LFI Ortho Module Transducer
analog to digital converter
Cosmic Microwave background
Feed Horn
[LFI meaning]: absolute calibration refers to the 0th order calibration for each channel, 1 single number, while the relative calibration refers to the component of the calibration that varies pointing period by pointing period.
System Level Test
Centre Spatial de Liège
Calibration and Performance Verification